The kilonova explosion that resulted when two neutron stars slammed into each other a billion light-years away turned out to be factory for rare heavy elements.
It's the first time the James Webb Space Telescope has probed such an event; and, in the aftermath of a colossal gamma-ray burst that emerged on 7 March 2023, the telescope's data revealed evidence of tellurium – a rare metal too heavy to be forged in the hearts of stars by the process of fusion.
There was also a suggestion of other metals, such as tungsten and selenium. The discovery, researchers say, confirms neutron star mergers as a source of heavy elements, an important piece of how our Universe makes material and spreads it across space.
"There are only a mere handful of known kilonovas, and this is the first time we have been able to look at the aftermath of a kilonova with the James Webb Space Telescope," says astrophysicist Andrew Levan of Radboud University, who led the analysis.
He adds, "Just over 150 years since Dmitri Mendeleev wrote down the periodic table of elements, we are now finally in a position to start filling in those last blanks of understanding where everything was made."
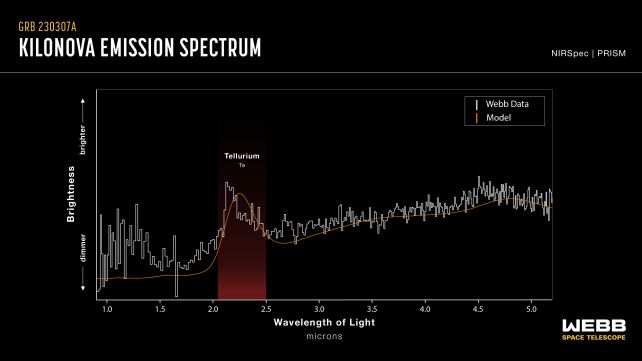
Stars are pretty wonderful things, really. They take the hydrogen that makes up most of Universe's visible matter, and smash its atoms together, over and over, to make heavier elements: hydrogen into helium, and then those heavier atoms into even heavier ones, all the way up to iron.
This is where stars' fusion engines run out of oomph, though. The fusion of iron into heavier elements requires a greater energy expenditure than it releases, setting the star on a path to go kaboom under the weight of its own gravity.
But this energetic blast can also generate a series of nuclear reactions in which atomic nuclei collide with loose neutrons to synthesize even heavier elements.
The reactions need to happen quickly enough that radioactive decay doesn't have a chance to occur before more neutrons are added to the nucleus. This means it needs to happen where there are a lot of free neutrons floating about – like within a supernova or kilonova. This particular nucleosynthesis process is known as the rapid neutron capture process, or r-process.
When two neutron stars were observed colliding for the first time in 2017, the aftermath confirmed that kilonovae produce r-process elements. Scientists detected the presence of strontium, the 38th element on the periodic table.
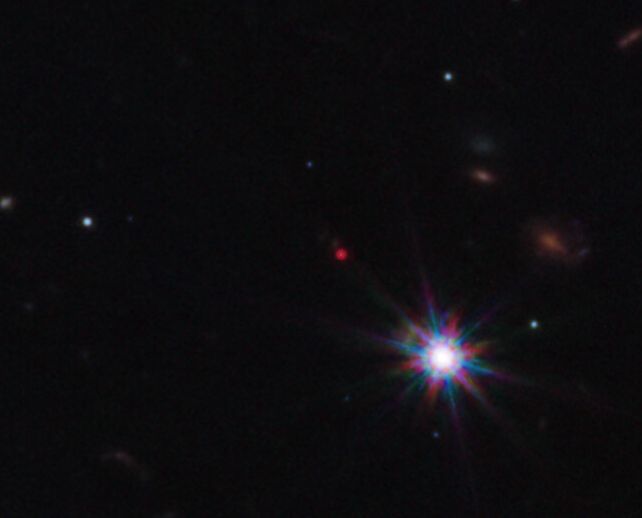
When a gamma-ray burst, named GRB230307A, was caught flaring in March of this year, scientists immediately tuned in for a closer look. GRB230307A was truly spectacular – one of the brightest gamma-ray bursts ever seen, 1,000 times brighter than typical and over a million times brighter than the entire Milky Way Galaxy.
It was also of an unusually long duration, at around 200 seconds. This long duration is thought to be a signature of a kilonova – supernova gamma-ray bursts are of a much shorter duration. Multi-wavelength observations confirmed it: the profile of the aftermath of the burst was consistent with a kilonova origin.
And since kilonovae are a known source of r-process elements, astronomers requested to take a look at the source of the explosion with the infrared JWST.
On 5 April, they turned the telescope to the glow, which by then had a significant infrared component, and collected spectra.
This data revealed the presence of tellurium, the 52nd element on the periodic table. That's pretty hefty. That means there are likely other r-process elements in the expanding ejecta from the neutron star collision, although more observations will be needed to confirm that.
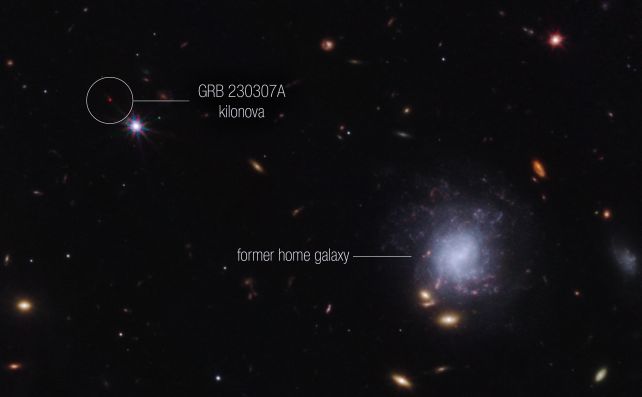
And it's worth noting that the explosion took place somewhere really weird: in intergalactic space, 120,000 light-years from the nearest galaxy. The researchers determined that the galaxy was probably where the two neutron stars had originated as normal, massive stars; when each went supernova some time in the past, one after the other, the force of the explosions was enough of a kick to yeet them clear out of the galaxy.
There's a lot more to be learned from this fascinating event, the researchers say.
"Until recently, we didn't think mergers could power gamma-ray bursts for more than two seconds," says astronomer Ben Gompertz of the University of Birmingham in the UK.
"Our next job is to find more of these long-lived mergers and develop a better understanding of what drives them – and whether even heavier elements are being created. This discovery has opened the door to a transformative understanding of our universe and how it works."
The research has been published in Nature.