Atoms may not have bones, but we still want to know how they are put together. These tiny particles are the basis on which all normal matter is built (including our bones), and understanding them helps us understand the larger Universe.
We currently use high-energy X-ray light to help us understand atoms and molecules and how they're arranged, catching diffracted beams to reconstruct their configurations in crystal form.
Now, scientists have used X-rays to characterize the properties of a single atom, showing that this technique can be used to understand matter at the level of its tiniest building blocks.
"Here," write an international team led by physicist Tolulope Ajayi of Ohio University and Argonne National Laboratory in the US, "we show that X-rays can be used to characterize the elemental and chemical state of just one atom."
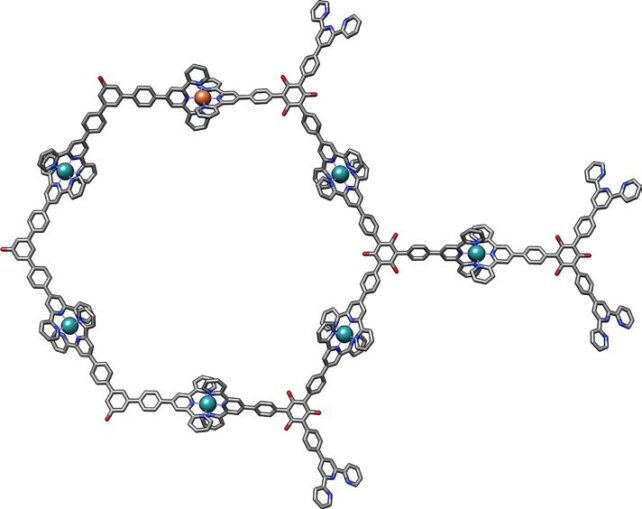
X-rays are considered a suitable probe for the characterization of materials on an atomic level because their wavelength distribution is comparable to an atom's size.
And there are several techniques for chucking X-rays at stuff to see how it's put together on really tiny scales.
One of those is synchrotron X-rays, in which electrons are accelerated along a circular track to the point they glow brightly with high energy light.
To try to resolve really fine scales, Ajayi and his colleagues used a technique that combines synchrotron X-rays with a microscopy technique for atomic-scale imaging called scanning tunneling microscopy. This employs an excellent sharp-tipped conducting probe that interacts with the electrons of test material in what is known as "quantum tunneling".
At very close proximities (like, half a nanometer), the precise position of an electron is uncertain, smearing it across the space between the material and the probe; the state of the atom can then be measured in the resulting current.
Together, the two techniques are known as synchrotron X-ray scanning tunneling microscopy (SX-STM). The amplified X-radiation excites the sample, and the needle-like detector collects the resulting photoelectrons. And it's an exciting technique that opens up some pretty incredible possibilities: Last year, the team published a paper on using SX-STM to rotate a single molecule.
This time, they went smaller still, attempting to measure the properties of a single iron atom. They separately created supramolecular assemblies, including iron and terbium ions inside a ring of atoms in what's referred to as a ligand. One iron and six rubidium atoms were linked with terpyridine ligands; terbium, oxygen, and bromine were linked using pyridine-2,6-dicarboxamide ligands.
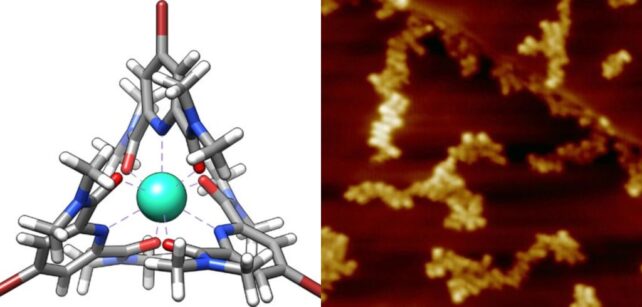
These samples were then subjected to SX-STM.
The light the detector receives isn't the same as the light beamed at the sample. Some wavelengths are absorbed by electrons in the atomic core, which means that there are some darker lines on the X-ray spectrum received.
These darker lines, the team found, are consistent with the wavelengths absorbed by iron and terbium, respectively. The absorption spectra could also be analyzed to determine the chemical states of these atoms.
For the iron atom, something interesting occurred. The X-ray signal could only be detected when the probe tip was located precisely above the iron atom in its supramolecular structure and at extremely close proximity.
This, the researchers say, confirms detection in the tunneling regime. Because tunneling is a quantum phenomenon, this has implications for studying quantum mechanics.
"Our work," the researchers write, "connects synchrotron X-rays with a quantum tunneling process and opens future X-rays experiments for simultaneous characterizations of elemental and chemical properties of materials at the ultimate single-atom limit."
That's probably at least as good as bones.
The research has been published in Nature.