Supermassive black holes are some of the heftiest objects in the Universe. At millions to billions of times the mass of the Sun, their evolution is challenging to explain.
And the higher mass range for these objects, particularly early in the history of the Universe, is even more challenging. These ultramassive black holes have masses over 10 billion Suns, and they're not just theoretical. A galaxy called J2157 spotted around 12.3 billion years ago hosted a black hole of 34 billion solar masses, while a galaxy called S5 0014+81 some 12.1 billion years ago had a 40 billion solar mass chonker.
If these black holes were just sitting there growing from feeding on material around them, there's no way, when the Universe was less than 10 percent of its current age, they would have had enough time to get that big.
Obviously, however, their existence isn't impossible. They're out there, after all. And a new simulation, using a powerful supercomputer to model the early Universe, has given us a means whereby these beasts can exist, without breaking our current cosmological models.
"We found that one possible formation channel for ultra-massive black holes is from the extreme merger of massive galaxies that are most likely to happen in the epoch of the 'cosmic noon'," explains astrophysicist Yueying Ni of the Harvard-Smithsonian Center for Astrophysics (CfA).
A slow growth by accretion is just one way in which black holes can gain mass. Another way, which we have actually observed in recent years, is the collision between two black holes. This is a sort of shortcut to gaining a large amount of mass, resulting in a black hole that is just short of the combined mass of the pre-merger objects, as a small percentage of mass escapes as gravitational energy during the merger.
To determine how ultramassive black holes can form in the early Universe, Ni and her colleagues used specially developed software called Astrid, designed to study the evolution of the Universe, including galaxy formation and mergers of supermassive black holes. They run Astrid on a supercomputer called Frontera at the Texas Advanced Computing Center.
A supercomputer is required, because you need a large volume of space to observe extreme outliers, like ultramassive black holes, and that, in turn, requires a large amount of computing power. But it paid off: by around 10 billion years ago, the researchers saw black holes of around 10 billion solar masses forming.
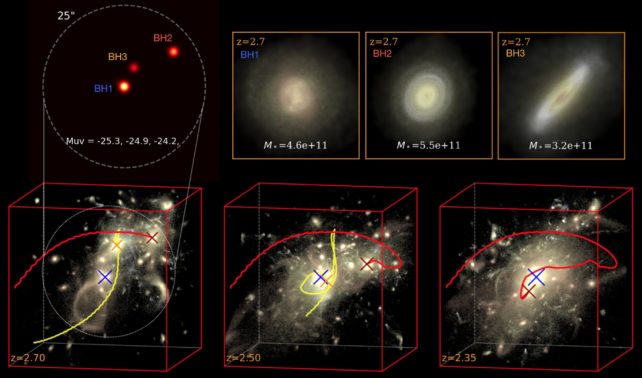
"What we found are three ultramassive black holes that assembled their mass during the cosmic noon, the time 11 billion years ago when star formation, active galactic nuclei (AGN), and supermassive black holes in general reach their peak activity," Ni says.
"In this epoch we spotted an extreme and relatively fast merger of three massive galaxies. Each of the galaxy masses is 10 times the mass of our own Milky Way, and a supermassive black hole sits in the center of each galaxy. Our findings show the possibility that these quasar triplet systems are the progenitor of those rare ultra-massive blackholes, after those triplets gravitationally interact and merge with each other."
We know that galaxies do sometimes collide and merge with each other – the Milky Way itself is a sort of Frankenstein's monster of smaller galaxies – and that these mergers can be three-way smash-ups. The Astrid simulation shows that this can occur in the early Universe, too, with high-mass quasars.
These are a class of galaxies with a hyperactive supermassive black hole at the center, actively guzzling down so much material that they blaze with light across billions of light-years, the brightest objects in the Universe.
When these galaxies merge, so too do their supermassive black holes, sinking towards the center of the newly combined massive galaxy to conduct an orbital dance that will eventually see a massive black hole coalescence. We don't know the rate at which these collisions occur – the frequency of the gravitational waves they emit is too low for our current detection range – but estimates suggest it happens quite a lot.
New advances in technology may mean we're much closer to finding observational evidence of these mergers, though. NASA's upcoming space-based Laser Interferometer Space Antenna (LISA) will be able to detect a much wider range of gravitational waves; and the powerful James Webb Space Telescope is even now peering into the distant Universe to unveil its secrets.
The team's findings and the Astrid simulations will be able to help scientists better interpret the JWST observations, and discover how the cosmic noon shaped the Universe we see around us today.
"This is an exciting time for astrophysicists," Ni says.
The research has been published in The Astrophysical Journal Letters.