On a basic level, a star is pretty simple. Gravity squeezes the star trying to collapse it, which causes the inner core to get extremely hot and dense. This triggers nuclear fusion, and the heat and pressure from that pushes back against gravity.
The two forces balance each other while a star is in its main sequence state. Easy peasy. But the details of how that works are extremely complex.
Modeling the interior of a star accurately requires sophisticated computer models, and even then it can be difficult to match a model to what we see on the surface of a star. Now a new computer simulation is helping to change that.
Although the internal pressure and gravitational weight of a star are generally in equilibrium, the flow of heat is not. All the heat and energy generated in a stellar core has to escape in time, and there are two general ways in which it happens.
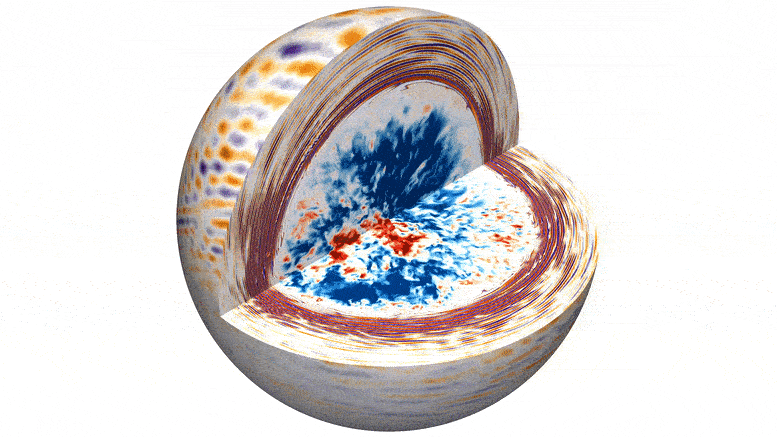
The first is through a radiative exchange. High-energy gamma rays scatter against nuclei in the core, gradually losing some energy as they migrate to the surface and escape. The interior of a star is so dense that this can take thousands of years.
The second method is through convective flow. Hot material near the center of a star tries to expand, pushing its way toward the surface. Meanwhile, cooler material near the surface condenses and sinks towards the core.
Together this creates a cyclic flow of material that transfers heat energy to the star's surface. This convection churns the interior of a star, and because of things such as viscosity and turbulent vortices, it is extremely difficult to model.
Stars generally have a radiative zone and a convective zone. The location and size of these zones depend on a star's mass. Small stars are almost entirely convective, while stars like the Sun have an inner radiative zone and an outer convective zone.
For massive stars, this is flipped, with an inner convective zone and an outer radiative one. One of the things we know about convection is that it can cause the surface of a star to fluctuate like a simmering pot of water. This in turn causes the overall brightness of a star to flicker slightly.
In this new study, the team has shown how convection regions in a star are connected to the way in which a star flickers. What they found was that sound waves rippling through a star are affected by convective flows, which in turn change the way a star flickers.
This means in principle we can study the interior of a star by observing its flicker of light, allowing astronomers to better understand stars.
Right now the flickers are too small for current telescopes to observe. But with larger and more sensitive telescopes we should be able to study them.
We are already able to study the effects of sound waves in the Sun, through what is known as helioseismology. In the coming decades, we should be able to do this with nearby stars.
This article was originally published by Universe Today. Read the original article.