Nearly three fourths of Earth is covered by oceans, making the planet look like a pale blue dot from space.
But Japanese researchers have made a compelling case that Earth's oceans were once green, in a study published in Nature.
The reason Earth's oceans may have looked different in the ancient past is to do with their chemistry and the evolution of photosynthesis. As a geology undergraduate student, I was taught about the importance of a type of rock deposit known as the banded iron formation in recording the planet's history.
Banded iron formations were deposited in the Archean and Paleoproterozoic eons, roughly between 3.8 and 1.8 billion years ago. Life back then was confined to one cell organisms in the oceans. The continents were a barren landscape of grey, brown and black rocks and sediments.
Rain falling on continental rocks dissolved iron which was then carried to the oceans by rivers. Other sources of iron were volcanoes on the ocean floor. This iron will become important later.
The Archaean eon was a time when Earth's atmosphere and ocean were devoid of gaseous oxygen, but also when the first organisms to generate energy from sunlight evolved. These organisms used anaerobic photosynthesis, meaning they can do photosynthesis in the absence of oxygen.
It triggered important changes as a byproduct of anaerobic photosynthesis is oxygen gas. Oxygen gas bound to iron in seawater. Oxygen only existed as a gas in the atmosphere once the seawater iron could neutralise no more oxygen.
Eventually, early photosynthesis led to the "great oxidation event", a major ecological turning point that made complex life on Earth possible. It marked the transition from a largely oxygen free Earth to one with large amounts of oxygen in the ocean and atmosphere.
The "bands" of different colours in banded iron formations record this shift with an alternation between deposits of iron deposited in the absence of oxygen and red oxidised iron.
The case for green oceans
The recent paper's case for green oceans in the Archaean eon starts with an observation: waters around the Japanese volcanic island of Iwo Jima have a greenish hue linked to a form of oxidised iron - Fe(III). Blue-green algae thrive in the green waters surrounding the island.
Despite their name, blue-green algae are primitive bacteria and not true algae. In the Archaean eon, the ancestors of modern blue-green algae evolved alongside other bacteria that use ferrous iron instead of water as the source of electrons for photosynthesis. This points to high levels of iron in the ocean.
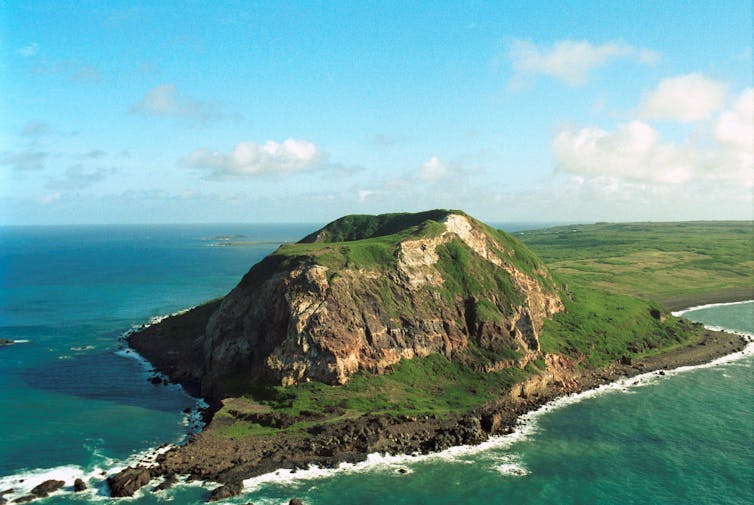
Photosynthetic organisms use pigments (mostly chlorophyll) in their cells to transform CO₂ into sugars using the energy of the sun. Chlorophyll gives plants their green colour. Blue-green algae are peculiar because they carry the common chlorophyll pigment, but also a second pigment called phycoerythrobilin (PEB).
In their paper, the researchers found that genetically engineered modern blue-green algae with PEB grow better in green waters. Although chlorophyll is great for photosynthesis in the spectra of light visible to us, PEB seems to be superior in green-light conditions.
Before the rise of photosynthesis and oxygen, Earth's oceans contained dissolved reduced iron (iron deposited in the absence of oxygen).
Oxygen released by the rise of photosynthesis in the Archean eon then led to oxidised iron in seawater. The paper's computer simulations also found oxygen released by early photosynthesis led to a high enough concentration of oxidised iron particles to turn the surface water green.
Once all iron in the ocean was oxidised, free oxygen (0₂) existed in Earth's oceans and atmosphere. So a major implication of the study is that pale-green dot worlds viewed from space are good candidates planets to harbour early photosynthetic life.
The changes in ocean chemistry were gradual. The Archaean period lasted 1.5 billion years. This is more than half of Earth's history. By comparison, the entire history of the rise and evolution of complex life represents about an eighth of Earth's history.
Almost certainly, the colour of the oceans changed gradually during this period and potentially oscillated. This could explain why blue-green algae evolved both forms of photosynthetic pigments. Chlorophyll is best for white light which is the type of sunlight we have today. Taking advantage of green and white light would have been an evolutionary advantage.
Could oceans change colour again?
The lesson from the recent Japanese paper is that the colour of our oceans are linked to water chemistry and the influence of life. We can imagine different ocean colours without borrowing too much from science fiction.
Purple oceans would be possible on Earth if the levels of sulphur were high. This could be linked to intense volcanic activity and low oxygen content in the atmosphere, which would lead to the dominance of purple sulphur bacteria.
Red oceans are also theoretically possible under intense tropical climates when red oxidised iron forms from the decay of rocks on the land and is carried to the oceans by rivers or winds. Or if a type of algae linked to "red tides" came to dominate the surface oceans.
These red algae are common in areas with intense concentration of fertiliser such as nitrogen. In the modern oceans, this tends to happen in coastline close to sewers.
As our sun ages, it will first become brighter leading to increased surface evaporation and intense UV light. This may favour purple sulphur bacteria living in deep waters without oxygen.
It will lead to more purple, brown, or green hues in coastal or stratified areas, with less deep blue colour in water as phytoplankton decline. Eventually, oceans will evaporate completely as the sun expands to encompass the orbit of Earth.
At geological timescales nothing is permanent and changes in the colour of our oceans are therefore inevitable.
Cédric M. John, Professor and Head of Data Science for the Environment and Sustainability, Queen Mary University of London
This article is republished from The Conversation under a Creative Commons license. Read the original article.