Inside your brain there is a map of every bedroom you've slept in. Every kitchen you've cooked in. Every city you've worked in, every country you've holidayed in. There's even a threadbare map of every Universe you've dreamt in.
Squeezing this vast trove of detailed information into a small tapestry of neurons is possible thanks to some very clever mathematics, according to a study on rat brains conducted by researchers in the US.
These newly discovered patterns of brain cell arrangement that embody the mental representation of physical space not only reveal how our brain stores certain kinds of data, but could provide insights into occasions where memory and mapping goes awry.
Enter a room for the first time, and your brain will hastily recruit neurons that will sketch out the space. These place cells aren't necessarily arranged in any way that mirrors the room, but their coordinated flashing still serves as a way to situate ourselves within a physical area.
Arranged into networks called place fields, those cells are repeatedly reorganized as we grow accustomed to the space, contributing to an increasingly enriched network of cells that ripple with correlated responses as the space around you becomes more familiar.
Just how this hierarchy of correlated activity develops and operates has so far been mostly speculative, at least from a mathematical point of view.
In a new study led by computational neurobiologist Tatyana Sharpee from the Salk Institute for Biological Studies, researchers investigated the activity of nerve cells in a part of the hippocampus of rats that's critical for their memory of spaces.
Using a previously devised method for studying place cells in rats as they run mazes, the researchers put a handful of adult rodents through their paces down a straight, 48-meter (157 foot) track, during which their neural activity was recorded as they completed runs.
There are a few ways a sequence of messages passed down through a network might be modeled, depending on their physical proximity or the ways different cells match in response.
An analysis of the hierarchy of signals flickering across a network of place cells in the rats was best modeled by a type of geometry described as hyperbolic, which – ironically enough – isn't the easiest geometry for our brains to picture.
Imagine, if you will, a typical office building with a boss at the top, sitting alone on a floor all to themself. The executives below the boss all have luxurious offices. Below them, middle managers squeeze into slightly smaller suites. Further down, a whole mass of workers crowd onto a floor full of cubicles.
This 'linear' hierarchy quickly runs out of room for each individual as you sink down through the floors and the additional departments add up.
However, an office tower constructed using hyperbolic geometry would have no problem accommodating new departments on the lower floors, which get exponentially larger, obeying a different set of rules on the angles intersecting lines form as they connect with different components.
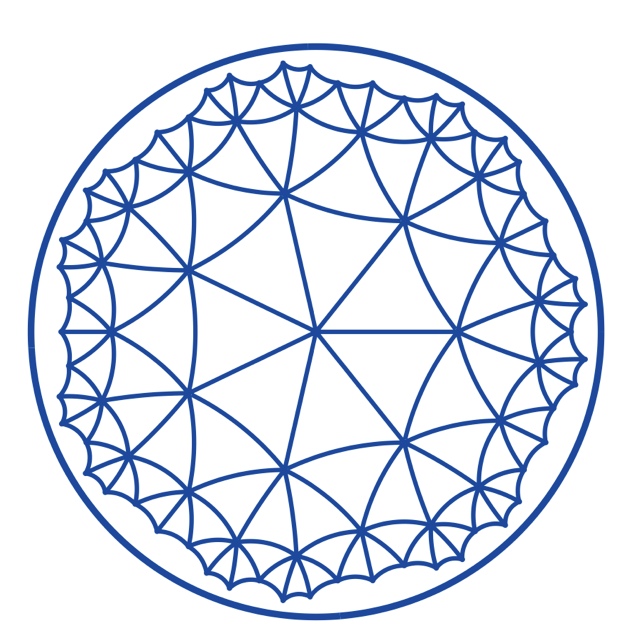
While we can use the above example to represent a hyperbolic hierarchy in flat space, in a full dimensional reality, those triangles would all be the same size (yes, trying to imagine this will hurt your brain). So, if this were a piece of material, the outer ends would curve with their excess circumference, like a floppy hat.
Hyperbolic hierarchies use similar mathematics to describe the relationships between different points of activity in a cascade of operations, allowing for a more efficient way to detail distances and objects in our minds as we picture ourselves in a space.
Here, the researchers observed the math in how small fields of place cells were quickly established when the rats were introduced to a new space, growing into more complex fields according to a logarithmic expansion as time went on.
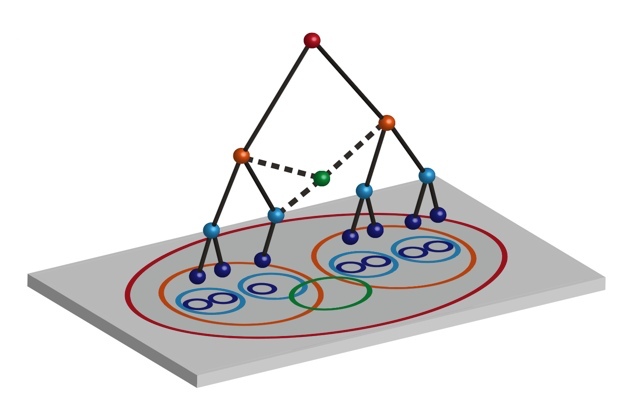
"Our study demonstrates that the brain does not always act in a linear manner. Instead, neural networks function along an expanding curve, which can be analyzed and understood using hyperbolic geometry and information theory," says Sharpee.
Recent studies found olfactory systems in biology also follow a hyperbolic hierarchy, allowing animals to categorize smells in far more complex and varied ways than a linear way of grouping scents would.
The researchers behind the new study argue hyperbolic representations in our spatial awareness adapt better to the reorganization that comes with a growing mental map, relying only on the information close at hand. Localizing the body in space is also more accurate than if the map developed according to a linear model.
Measuring similar effects in humans could inform models on disease, especially in fields of neurology involved with memory and spatial awareness.
On a more poetic level, there's a beauty in knowing the expansion of our mental Universe reflects the infinite expansion of our physical one. While all signs so far point to our Universe having a flat shape, there are models that speculate whether the total geometry of space-time might yet have a subtle curvature to it.
"You would think that hyperbolic geometry only applies on a cosmic scale, but that is not true," says Sharpee.
"Our brains work much slower than the speed of light, which could be a reason that hyperbolic effects are observed on graspable spaces instead of astronomical ones. Next, we would like to learn more about how these dynamic hyperbolic representations in the brain grow, interact, and communicate with one another."
This research was published in Nature Neuroscience.