Using an anvil made of diamond, physicists have successfully squeezed iron into the form we think it has deep in the center of Earth.
It's called hexaferrum, or epsilon iron (ϵ-Fe), and it's only stable at extremely high pressures. Scientists think the majority of the iron in Earth's core takes this form, and a detailed understanding of its properties could help us understand why the very center of our planet seems to have directional variations in its texture – a property known as anisotropy.
There's just one problem in this quest to understand Earth's core. Here on the surface, in a nice, relatively low atmospheric pressure regime, conditions in the core are difficult to replicate. But we can create high-pressure conditions for brief pulses of time, using diamond anvils and heat.
"We report here the synthesis of ϵ-Fe single crystals in diamond anvil cells and subsequent measurement of single-crystal elastic constants of this phase up to 32 GPa at 300 Kelvin with inelastic X-ray scattering," write a team led by physicist Agnès Dewaele of the University of Paris-Saclay in France.
The challenge lay in converting the atmospheric pressure phase of iron called ferrite, or alpha iron. Usually, when high pressure is applied to ferrite in an attempt to crush it into hexaferrum, it fractures into tiny crystals that are unsuitable for detailed analysis, which frustrates efforts to study its elastic properties.
So, Dewaele and her colleagues approached the problem stepwise. They placed crystals of ferrite in a diamond anvil in a vacuum heater, and increased the pressure to 7 gigapascals (that's around 70,000 times the atmospheric pressure at sea level) and the temperature to 800 Kelvin (527 degrees Celsius, or 980 Fahrenheit).
This produced an intermediate phase of iron that occurs at high temperatures in atmospheric conditions called austenite, or gamma iron. Austenite has a different structure to ferrite, and the austenite crystals the team made changed into the hexaferrum phase far more smoothly at pressures between 15 and 33 gigapascals at 300 Kelvin.
Then, they used a synchrotron beamline at the European Synchrotron Radiation Facility to probe the hexaferrum and analyze its properties.
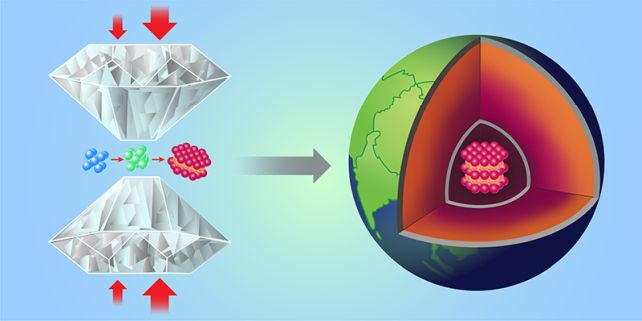
What we know of Earth's core is largely reconstructed based on seismic data. Acoustic waves created by planetary tremors propagate differently through different materials; this is how we know that Earth's core is layered like a jawbreaker.
But for a more detailed understanding, we need to know what the material in the core actually is, and how it responds to acoustic waves. The work of Dewaele and her team showed that hexaferrum's elasticity is directionally dependent; waves propagate faster along one particular axis.
This anisotropy persists during pressure changes, too, which suggests that it's also how hexaferrum behaves in the up to 360 gigapascal environment of the inner core. This is consistent with observations of how seismic waves travel through the planet.
The findings suggest that the team's techniques could make an excellent probe for understanding the extreme conditions at the center of our world.
The research has been published in Physical Review Letters.