Given the right circumstances, it is possible for sound to travel through a perfect vacuum. Now two physicists have worked out what those conditions need to be.
Zhuoran Geng and Ilari Maasilta of the University of Jyväskylä in Finland say their findings represent the first rigorous proof of complete acoustic tunneling in a vacuum.
To achieve it, you'll need two piezoelectric materials, which are capable of turning movements into voltages (and vice versa). The objects need to be separated by a gap that's smaller than the wavelength of the sound you want to send, which will then completely jump – or 'tunnel' – across that space.
We've known about acoustic wave tunneling since the 1960s, but scientists have only begun to investigate the phenomenon relatively recently, which means we don't yet have a very good understanding of how it works.
Geng and Maasilta have been working on fixing that, first by describing a formalism for the study of acoustic tunneling, and now by applying it.
In order to propagate, sound requires a medium to travel through. Sound is generated by vibrations, which causes atoms and molecules in the medium to vibrate; that vibration is passed on to adjacent particles. We sense these vibrations via a sensitive membrane in our ears.
A perfect vacuum is a complete absence of a medium. Since there are no particles to vibrate, sound shouldn't be able to propagate.
But there are loopholes. What qualifies as a vacuum can still buzz with electrical fields, which makes piezoelectric crystals an intriguing material for the study of sound across otherwise empty spaces.
These are materials that convert mechanical energy into electrical energy, and vice versa. In other words, if you place a mechanical stress on the crystal, it will produce an electric field. And if you expose the crystal to an electrical field, the crystal will deform. That's known as the inverse piezoelectric effect.
OK this is where it gets fun. A sound vibration exerts mechanical stress. Using zinc oxide as their piezoelectric crystals, Geng and Maasilta found that a crystal can convert this stress into an electrical field if certain conditions are met.
If there is a second crystal within range of the first, it can convert the electrical energy back into mechanical energy – et voila, the sound wave has traversed the vacuum. In order to do this, the two crystals have to be separated by a gap no wider than the length of the initial acoustic wave.
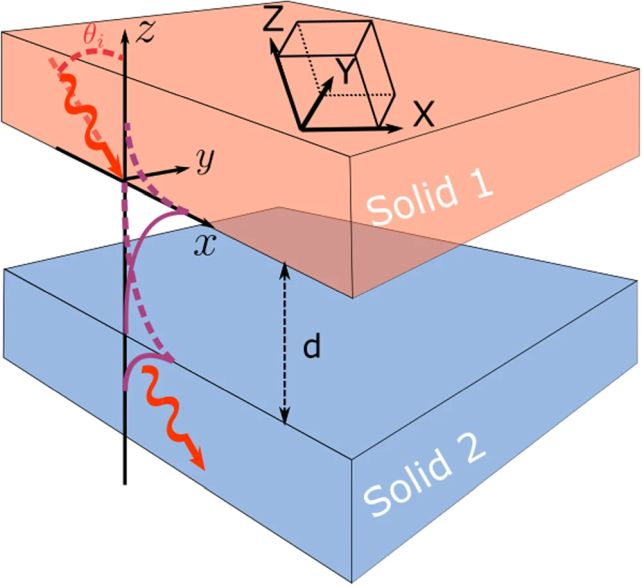
And the effect scales with frequency. As long as the vacuum gap is scaled accordingly, even ultrasound and hypersound frequencies can tunnel through the vacuum between the two crystals.
Because the phenomenon is analogous to the quantum mechanical effect of tunneling, the results of the research could help scientists study quantum information science, as well as other areas of physics.
"In most cases the effect is small, but we also found situations where the full energy of the wave jumps across the vacuum with 100 percent efficiency, without any reflections," Maasilta says.
"As such, the phenomenon could find applications in microelectromechanical components (MEMS, smartphone technology) and in the control of heat."
The research has been published in Communications Physics.