Odd things happen inside planets, where familiar materials are subjected to extreme pressures and heat.
Iron atoms probably dance within Earth's solid inner core, and hot, black, heavy ice – that's both solid and liquid at the same time – likely forms within the water-rich gas giants, Uranus and Neptune.
Five years ago, scientists recreated this exotic ice, called superionic ice, for the first time in lab experiments; and four years ago they confirmed its existence and crystalline structure.
Then just last year, researchers at several universities in the United States and the Stanford Linear Accelerator Center laboratory in California (SLAC) discovered a new phase of superionic ice.
Their discovery deepens our understanding as to why Uranus and Neptune have such off-kilter magnetic fields with multiple poles.
From our earthly surrounds, you'd be forgiven for thinking water is a simple, elbow-shaped molecule made up of one oxygen atom linked to two hydrogens that settle into a fixed position when water freezes.
Superionic ice is strangely different, and yet it may be among the most abundant forms of water in the Universe – presumed to fill not only the interiors of Uranus, Neptune, but also similar exoplanets.
These planets have extreme pressures of 2 million times the Earth's atmosphere, and interiors as hot as the surface of the Sun – which is where water gets weird.
Scientists confirmed in 2019 what physicists had predicted back in 1988: a structure where the oxygen atoms in superionic ice are locked in a solid cubic lattice, while the ionized hydrogen atoms are let loose, flowing through that lattice like electrons through metals.
This gives superionic ice its conductive properties. It also raises its melting point such that the frozen water remains solid at blistering temperatures.
In this latest study, physicist Arianna Gleason of Stanford University and colleagues bombarded thin slivers of water, sandwiched between two diamond layers, with some ridiculously powerful lasers.
Successive shockwaves raised the pressure to 200 GPa (2 million atmospheres) and temperatures up to about 5,000 K (8,500 °F) – hotter than the temperatures of the 2019 experiments, but at lower pressures.
"Recent discoveries of water-rich Neptune-like exoplanets require a more detailed understanding of the phase diagram of [water] at pressure–temperature conditions relevant to their planetary interiors," Gleason and colleagues explain in their paper, from January 2022.
X-ray diffraction then revealed the hot, dense ice's crystal structure, despite the pressure and temperature conditions only being maintained for a fraction of a second.
The resulting diffraction patterns confirmed the ice crystals were in fact a new phase distinct from superionic ice observed in 2019. The newly discovered superionic ice, Ice XIX, has a body-centered cubic structure and increased conductivity compared to its predecessor from 2019, Ice XVIII.
Conductivity is important here because moving charged particles generate magnetic fields. This is the basis of dynamo theory, which describes how churning conductive fluids, such as Earth's mantle or inside another celestial body, give rise to magnetic fields.
If more of a Neptune-like ice giant's insides were taken up by a mushy solid, and less of by a swirling liquid, then it would change the kind of magnetic field produced.
And if towards its core that planet had two superionic layers of differing conductivity, as Gleason and colleagues suggest Neptune might contain, then the magnetic field generated by the outer liquid layer would interact with each of them differently, making things stranger still.
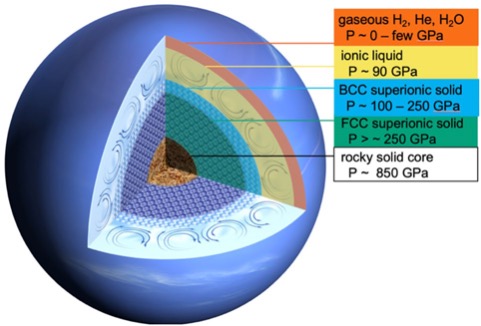
Gleason and colleagues conclude the enhanced conductivity of a layer of superionic ice akin to Ice XIX would promote the generation of wonky, multipolar magnetic fields like those emanating from Uranus and Neptune.
If so, it would be a satisfying result more than 30 years after NASA's Voyager II space probe, launched in 1977, flew by our Solar System's two ice giants and measured their highly unusual magnetic fields.
The study was published in Scientific Reports.