There are places in the Universe where matter becomes so wildly distorted that magnetism grows into an unimaginable force. Known as magnetars, the gravitationally compacted cores of these highly dynamic neutron stars concentrate magnetic fields to a strength of around 100 trillion gauss.
Yet there could be zones here on Earth where tiny pockets of magnetism flicker with strengths that far exceed even these cosmic monstrosities.
An analysis of particle interactions at the Relativistic Heavy Ion Collider (RHIC) at the US Department of Energy's (DOE) Brookhaven National Laboratory has found traces of record-breaking magnetic fields imprinted on the spray of material shed by crashing together nuclei of various heavy ions.
By measuring the shrapnel of even smaller quark and gluon particles set free by the off-center collisions, physicists have gained insights into the forces at work deep inside atoms.
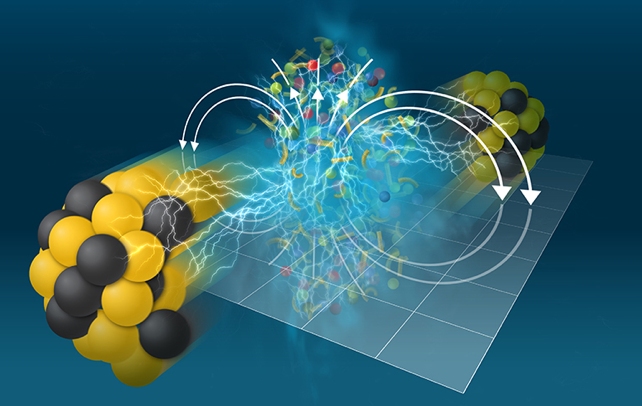
"This is the first measurement of how the magnetic field interacts with the quark-gluon plasma (QGP)," says Diyu Shen, a physicist at the Solenoidal Tracker at RHIC (STAR) collaboration at the DOE.
Quarks are fundamental particles that flicker in and out of existence in quantum blizzards, their interactions governed by briefly lived gluon particles that bind the quark and antiquark tempests into the protons and neutrons that make up all atoms.
Knowing just how quarks and antiquarks duck and dive in their short lifetimes inside nuclear particles helps physicists better understand the construction of matter from the ground up, yet the space at the heart of an atom is no place for the faint-hearted.
While it's theoretically possible to map the activity of quarks and their oppositely charged antiquark twins using something known as the chiral magnetic effect, in practice the electromagnetic field within a fog of exposed quarks and gluons is too short-lived to catch sight of, rapidly succumbing to the flow of competitive currents.
One situation in which physicists thought a handy magnetic field might be generated was a collision between heavy nuclei not perfectly on-center.
By clipping one another, the protons within the massive bundles would be sent spiraling in a charged swirl that would result in a powerful eddy of magnetism – so powerful, they could deliver more gauss than a quaking neutron star.
"Those fast-moving positive charges should generate a very strong magnetic field, predicted to be 1018 gauss," says STAR physicist Gang Wang.
"This is probably the strongest magnetic field in our Universe."
That would make these flashes of magnetism 10,000 times stronger than the most powerful magnetar, and 10 quadrillion times stronger than the 100 gauss of a typical fridge magnet.
Where magnetars could churn out their magnetic maelstroms for tens of thousands of years, these proton-induced bursts of magnetism would last a mere ten millionths of a billionth of a billionth of a second, making any glimpse of the field itself impossible.
Yet its presence would still be felt by the charged quarks let loose by the collision.
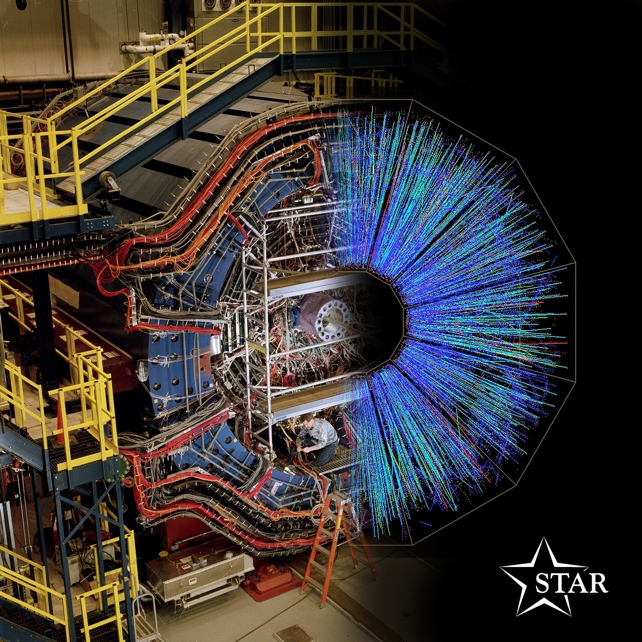
By throwing gold nuclei at one another at various energies, along with mutual collisions of ruthenium and zirconium, the researchers were able to sift through the remnants and identify paths taken by particles that signified the magnetic field's presence.
Knowing this, they could measure the distribution of particles in a way that provided important details on the quark-gluon plasma's electrical conductivity.
"We can infer the value of the conductivity from our measurement of the collective motion," says Shen.
"The extent to which the particles are deflected relates directly to the strength of the electromagnetic field and the conductivity in the QGP – and no one has measured the conductivity of QGP before."
This research was published in Physical Review X.