Microbial life in Yellowstone's Lower Geyser Basin may hold clues to the evolution of life's exploitation of oxygen, according to a recent analysis by researchers from Montana State University.
The inhabitants of the basin's Octopus and Conch Springs live in kelp-like, gelatinous 'streamer' structures that wiggle furiously in superheated currents, which hover around 88 degrees Celsius (190 degrees Farenheit). Genetically similar to ancient bacteria and archaea, their existence is a window into the primordial soup from which life emerged.
While these microbial communities share many traits, the springs' environments are different in a few fundamental ways.
Octopus Spring has far higher levels (about 20 micromolar) of dissolved oxygen than the neighboring Conch Spring, which with less than 1 micromolar of dissolved oxygen has almost none.
Meanwhile, Conch Spring has far more of the highly toxic dissolved sulfide (more than 120 micromolar) than Octopus Spring (less than 2-3 micromolar).
These differences in chemistry means comparisons between the communities in each spring can help us understand how life survived before – and throughout – the Great Oxidation Event (GOE) that flooded Earth's nearly oxygen-free atmosphere with our favorite gas around 2.5 billion years ago.
Earth's earliest microbes no doubt developed ways to weave traces of oxygen into their biochemistry prior, yet the appearance of highly-reactive molecular oxygen would have demanded the evolution of whole new defense tactics.
What's more, in high concentrations sulfides can block up respiration machinery in today's aerobic organisms, further posing questions on how ancient life in hot springs may have evolved to combat and make use of rising oxygen levels.
With their drastically different levels of oxygen and sulfide, the springs can be considered proxies for life on either side of the GOE, giving researchers the perfect grounds to dig for clues on this vital transition.
The study was led by geomicrobiologist Bill Inskeep, who has been studying Yellowstone's heat-loving microbes since 1999.
"It would be very difficult to reproduce this kind of an experiment in the laboratory; imagine trying to create hot-water streams with just the right amounts of oxygen and sulfide," Inskeep says.
"And that's what's so nice about studying these environments. We can make these observations in the exact geochemical conditions that these organisms need to thrive."
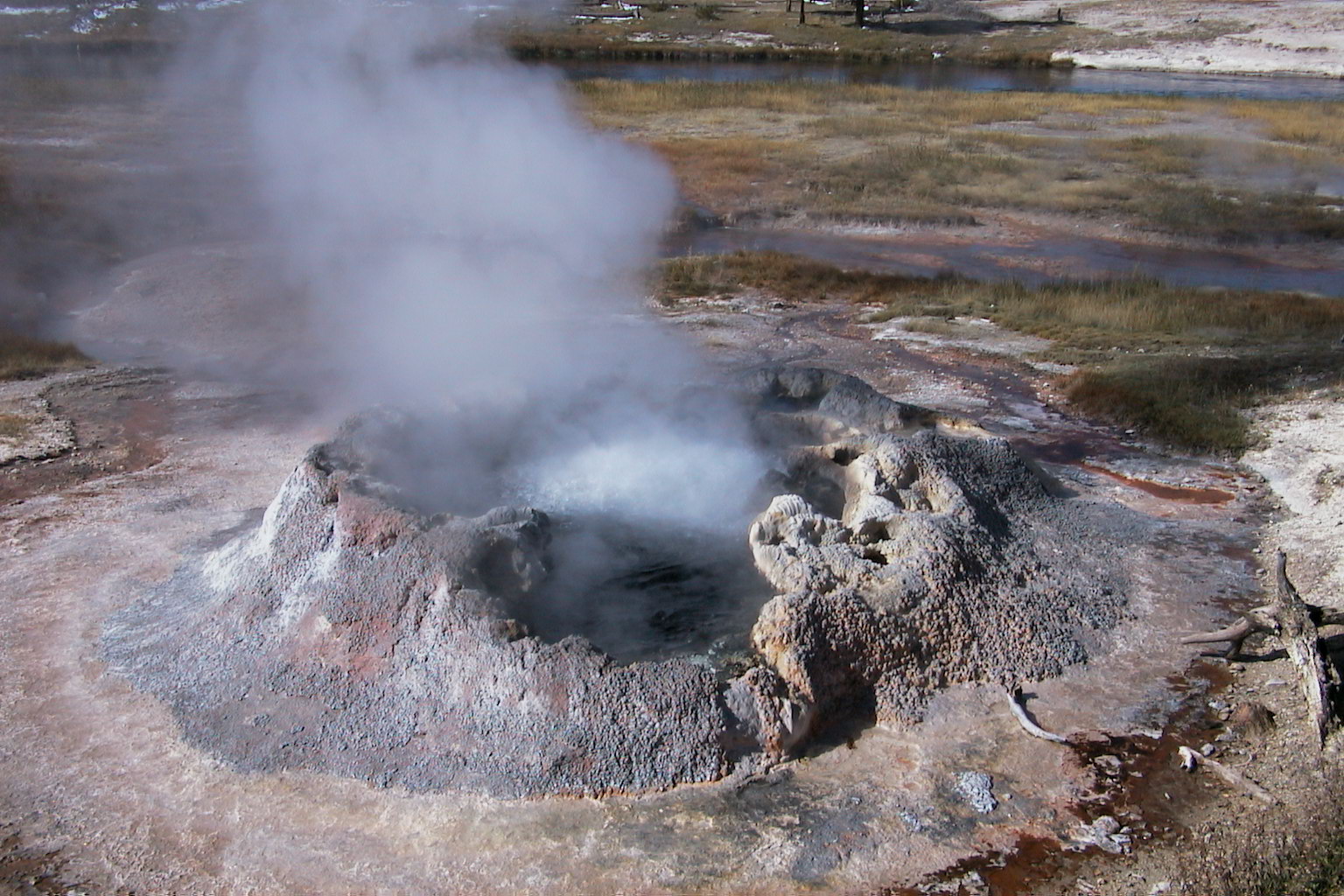
By analyzing the microbe samples' genes and their products, the team could compare microbial diversity and respiratory activity in the two springs.
"The streamer communities are large enough structures to be visible with the naked eye," Inskeep wrote for Nature's Research Communities.
"This physical reality informs us that gas exchange is necessary for optimum growth, which is also further influenced by the rapid oscillation of large filamentous structures that create turbulence and likely increase rates of gas exchange with the atmosphere."
In the higher-oxygen environment of Octopus Spring, there was a greater diversity of the oxygen-hungry microbes that must eat other organisms to survive (rather than making their own food through chemical reactions, as other microbes sometimes do).
In fact, life in Octopus Spring was more diverse in general. And nearly all the microorganisms there had active genes for oxygen respiration.
But even in sulfidic, asphyxiating Conch Spring, which had far lower microbial diversity within its "very snotty" streamers, the potential for oxygen use seems to lie latent.
A variety of enzymes that defuse oxygen by adding hydrogen were found in among the most common populations, and were produced in both springs. Significantly, the enzymes with the highest affinity for oxygen were only expressed in the habitat with high concentrations of sulfide.
"These high-affinity oxygenases are active at nanomolar levels of oxygen, and explain the high transcription observed under sulfidic conditions of Conch Spring," Inskeep wrote.
Their results showed that these genetically ancient, heat-loving microbes respire at oxygen levels usually deemed too low to support such a task in conditions we'd today think of as too toxic. And if they can do it, why not life forms that emerged before the Great Oxidation Event?
There may not have been much to work with in the early days of Earth, but some early aerobic metabolisms may have scraped by on just enough oxygen to pass on genes that would one day thrive in an oxygen-rich atmosphere.
So next time you enjoy a deep breath, remember it wasn't always so easy.
The study was published in Nature Communications.